Exploring Green Hydrogen Pathways Beyond Solar: Achieving the ₹100/kg Benchmark
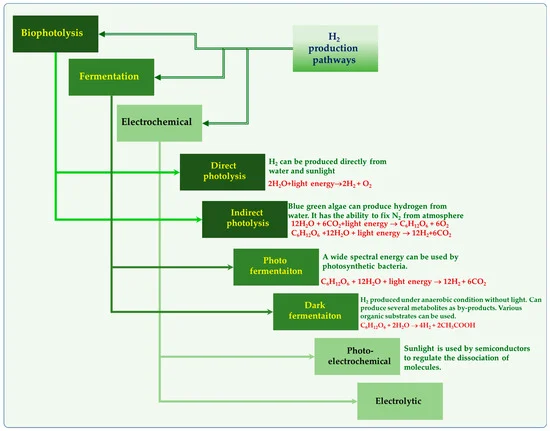
Image : Sarkar, O., Modestra, J. A., Rova, U., Christakopoulos, P., & Matsakas, L. (2023). Waste-Derived Renewable Hydrogen and Methane: Towards a Potential Energy Transition Solution. Fermentation, 9(4), 368. https://doi.org/10.3390/fermentation9040368
Introduction: Green hydrogen has emerged as a cornerstone in the global transition to a low-carbon economy. While solar-powered hydrogen production through electrolysis remains one of the most explored methods, achieving a cost-effective price benchmark of ₹100/kg requires innovation across diverse production pathways. This article delves into alternative methods of green hydrogen production and the economic considerations that can drive cost efficiency while promoting sustainability.
1. Current Status of Green Hydrogen Production:
Solar-powered electrolysis, where electricity derived from solar power is used to split water into hydrogen and oxygen, has garnered significant attention. Despite its potential, the cost of solar-driven hydrogen is still relatively high, owing to factors such as infrastructure investments and energy conversion inefficiencies.
However, the future of green hydrogen hinges on expanding the scope of production methods, particularly focusing on more efficient, sustainable, and cost-effective alternatives.
2. Alternative Green Hydrogen Pathways:
a. Biological Hydrogen Production:
- Direct Photolysis: In this process, microorganisms like algae utilize sunlight to split water molecules and release hydrogen. While the concept is similar to electrolysis, the reliance on biological organisms could lower operational costs in some regions. However, scalability remains a challenge due to the slow hydrogen production rates.Economic Consideration: Direct photolysis can be cost-effective when optimized in localized small-scale systems. However, current research needs to focus on improving yields to make it commercially viable.
- Dark Fermentation: Under anaerobic conditions, certain bacteria can produce hydrogen from organic materials such as waste or biomass. This method not only produces hydrogen but also offers a dual advantage of managing organic waste.Economic Consideration: Using waste as a feedstock for hydrogen production presents a low-cost alternative. With advancements in microbial engineering, dark fermentation can potentially contribute to cost reductions by utilizing existing waste streams, thus lowering feedstock costs.
- Photofermentation: Similar to dark fermentation, this method uses photosynthetic bacteria to convert organic substrates into hydrogen. This pathway allows the use of a wide spectrum of light energy, potentially increasing efficiency.Economic Consideration: The versatility of this pathway to utilize various organic materials could significantly reduce costs if paired with waste management systems, leading to a dual-purpose process that cuts both waste disposal and hydrogen production costs.
b. Electrochemical Pathways:
- Photo-electrochemical Hydrogen Production: In this method, sunlight is used directly to drive the electrochemical reaction that splits water molecules into hydrogen and oxygen. By using semiconductor materials, this process can make hydrogen production more energy-efficient.Economic Consideration: The economic viability of photo-electrochemical processes depends on advancements in semiconductor technology to improve efficiency and reduce costs. With large-scale adoption, the per-unit cost of materials can be reduced, lowering the overall cost of hydrogen production.
- Electrolytic Hydrogen from Renewable Energy: Although solar electrolysis is widely discussed, integrating other renewable energy sources, such as wind and hydropower, could diversify and stabilize hydrogen production costs. In regions with consistent wind or hydropower, this can ensure continuous operation, thus reducing downtime and improving cost efficiency.Economic Consideration: By blending multiple renewable sources, the cost of electricity used in electrolysis could be balanced, leading to a more predictable and lower price of hydrogen production.
3. Waste-Derived Hydrogen:
Waste materials, such as agricultural residues, food waste, and industrial by-products, offer another viable feedstock for hydrogen production. Using microbial electrolysis cells (MECs), biohydrogen can be generated through the biocatalysis of electroactive microbes, converting organic waste into hydrogen and methane.
Economic Consideration: The dual benefit of waste management and energy generation positions this method as highly cost-effective. Scaling up biohydrogen production from waste materials could achieve significant cost reductions, potentially approaching the ₹100/kg benchmark, especially with government incentives for waste-to-energy projects.
4. Economic Outlook and Pathway Synergies:
Achieving the ₹100/kg hydrogen price will require not only advancements in individual pathways but also integrated systems that combine multiple production methods. For example, a hybrid system could utilize dark fermentation to produce hydrogen from waste, coupled with photo-electrochemical methods for additional production. By leveraging local resources, such as organic waste or renewable electricity, a synergistic approach could ensure both cost efficiency and scalability.
5. Techno-Economic Challenges:
Scaling hydrogen production from emerging pathways comes with its own challenges. The cost of infrastructure, purification, storage, and distribution needs to be minimized to achieve price competitiveness with conventional fossil fuels. Research into improving yields, process efficiencies, and reducing capital expenditures (CAPEX) will be critical in meeting this target.
Key Focus Areas for Cost Reduction:
- Feedstock Utilization: Waste-derived hydrogen offers the most promise for cost-effective production by using inexpensive or even negative-cost feedstocks.
- Process Efficiency: Enhancements in microbial processes, light absorption in biological routes, and advancements in electrolyzer technology are necessary to drive down costs.
- Storage and Transportation: Innovations in solid-state hydrogen storage and compression techniques will also play a key role in achieving economic viability.
Conclusion:
The race to produce green hydrogen at an affordable rate is intensifying. While solar-powered electrolysis remains a significant focus, looking beyond solar and exploring diverse hydrogen production pathways will be key to meeting economic targets. Biological and electrochemical methods, along with waste-derived hydrogen, offer promising avenues that could bring hydrogen prices closer to the ₹100/kg benchmark, driving India’s green hydrogen ambitions forward.